Introduction
Aquaculture is a rapidly growing industry worldwide, from which people derive about 50% of their animal protein requirements.1 As the Food and Agriculture Organization reported in 2020, aquaculture production in 2030, which provides about 1/3 of the world’s aquaculture resources, is estimated to rise to 53%.2 In addition to fish, species such as carp, tilapia, and trout and aquatic organisms such as oysters, clams, and shrimps are grown in aquaculture in fresh and marine waters.3
However, aquaculture suffers from heavy financial losses every year globally because of viral, fungal, parasitic, and bacterial disease outbreaks at any stage of the breeding process.4–7 The same bacterial pathogens detected disease agents in aquatic organisms: Gram-negative Aeromonas salmonicida, A. hydrophila, Pseudomonas plecoglossicida, Edwardsiella tarda, E. piscicida, E. ictaluri, Vibrio spp. (V. harveyi, V. parahaemolyticus, V. anguillarum, V. splendidus, V. alginolyticus, V. coralliilyticus), Flavobacterium columnare, F. psychrophilum, Yersinia ruckeri and Gram-positive Lactococcus garvieae, Renibacterium salmoninarum, Streptococcus iniae, Mycobacterium species.8,9 These bacterial pathogens that are easily transmitted through water in case of external stressors, including intensive stock densities, inadequate nutrition, build-up of toxic chemicals, poor water quality, and low oxygenation, can therefore infect many aquatic organisms.
Various strategies, including probiotics, prebiotics, immunostimulants, and vaccination, have increased fish defense and prevented bacterial diseases.10,11 However, vaccine administration methods and routes vary depending on species, quantity, size of organisms, pathogens, temperature, and environment.12,13 Therefore, vaccination becomes a tedious job for large-scale aquaculture systems. Also, vaccines may not be as effective against larvae and invertebrates without a robust immune system.14 Of the biocides, malachite green is used in the treatment of protozoal and fungal infections, while formaldehyde and formalin solutions are prophylactic disinfectants for eggs and larval development.
The use of amoxicillin, oxytetracycline, sulfonamides, tetracyclines, nitrofurans, fluoroquinolones, and florfenicol among antibiotics as a therapeutic agent is also the most preferred method to inhibit the growth of bacteria and stop heavy mortalities during outbreaks of infectious bacterial diseases in aquaculture and fisheries.8,13,15 Although they are rapid, effective and commenly used for bacterial infection of aquaculture and agriculture, antibiotics generally target both pathogenic and non-pathogenic microflora of the environment. In additional, their long-term and heavy use caused a number of unfavourable impacts such as accumulation and toxicity in organisms, occurence of antibiotic-resistant bacterial strains and suppression of the immune response of the host, thus increasing the ineffectiveness of antibiotic treatments.9,11,13,16 The above disadvantages of antibiotics have prompted the development of species-specific, eco-friendly and less expensive way to prevent bacterial infectious diseases in sustainable aquaculture.3,7 In search of alternative tool or a possible solution, the use of bacteriophages seems to be very promising and appropriate strategy as approached “post-antibiotic era” as the World Health Organisation (WHO) announced in different diseases of animals.17–22
This review mainly focuses on and summarizes extensive research literature in the last 40 years and future directions, with many different bacteriophages and its applications and outcomes as alternative ways, due to overuse of antibiotics, to prevent, control and treat diseases in aquatic organisms (such as crustaceans, molluscs and fish) and their environment.
Phage morphology
The bacteriophages, also known as phages (meant to imply “eat” or “devour” in Greek), are very small bacterial viruses that can range in size from 20 to 200 nm, are host-specific, and can only infect and kill targeted bacteria, without harming the surrounding microbiota and animal or plant cells.23–25 Diverse phages can be found in all environments that are abundant in nature, such as river and seawater, sediments, soil, sewage, and food products, and were also readily detected in human and animal feces/urine.26–28
The taxonomy and three-dimensional structure of typical phage morphology is well defined. It is classified according to their general morphology, the presence of outer envelope and lipid structures, and the type of genome that is in the form of ssRNA, dsRNA, ssDNA and dsDNA.17,29–31 According to this approach, the International Committee for Taxonomy of Viruses (ICTV) identified 14 distinct and well-characterized phage families: Myoviridae, Podoviridae, Siphoviridae, Microviridae, Inoviridae, Herelleviridae, and Ackermannviridae, as shown in Table 1. Table 1 has been prepared according to information from Acherman32,33 and Sharp et al.34

Table 1.Morphology and genome characteristics of the fourteen phage families
The phage genome is enclosed in a protein capsid head (e.g., filamentous, helical, icosahedral, pleomorphic, and polyhedral), a tail with spiral sheath and tail fibers and surface receptors responsible for recognizing specific host bacterial molecules and attaches themselves to the cell’s surface.35,36 These phages, which cannot perform their molecular replication under normal conditions, now use the host mechanism to reproduce themselves after their genome is injected into the bacterial host. They take over the bacterial biosynthesis control mechanism and command the bacterial host to produce different viral proteins and release progeny and phages that can continue to infect other hosts.
Phage life cycle
Phages can multiply and propagate by infecting bacteria in 2 paths: 1) lytic life cycle (virulent) and 2) lysogenic life cycle (temperate-dormant). The first phage cycle, which lasts between 20 minutes and 2 hours, begins when phages attach to the host, integrate their genetic material, and continue to multiply to produce viral progeny. The virulent phages will control the host’s protein. This cycle results in the secretion of lysins and holins enzymes by phages, lysis of the host bacterial cell membrane, and releasing the newly formed progeny virions into the environment. Afterward, the new progenies infect different host bacteria. The lysogenic (temperate) phages, in contrast, attach their genome to the host’s and remain in a dormant and stable stage for a long time until environmental conditions are favorable for the rapid growth of new prophages.36–42 Therefore, lytic phages that proliferate exponentially and damage the pathogenic host in any case of antibiotic resistance status are more amenable to developing therapeutic intent.
Phage history and their potential applications
Phages therapy first came onto the scientific domain about a hundred years ago after finding by Twort43 and d’Herelle,44 respectively.23,40,45–48 Phage has been used successfully to treat severe hemorrhagic Shigella dysentery among French troops patients and against cholera by Vibrio cholera in India.30,31,49,50 Bruynoghe and Maisin51 reported phage therapy treatment of staphylococcal skin disease. The first commercial phage in history was the anticholine phage, successfully used to control the epidemic that threatened the southeastern regions of the Soviet Union (SSCB) and then Georgia in 1931.52 In the 1930s and 1940s, the phage therapy application against mixed bacteria caused by Clostridium perfringens, Staphylococcus, Streptococcus, Escherichia coli and Proteus species were tested in Poland, Belarus, Georgia, Russia, Ukraine, and Azerbaijan.23,53 Concurrent with the advent of commercial antibiotics in the 1940s, there was a huge decline in using phages as therapeutic agents in Western countries and the United States. However, in the period from the 1950s to late 1970s, the SSCB and in East Europe continued using the phage treatment against S. typhi and S. paratyphi and phage for prophylaxis in the fast spread of infections occurred such as military and schools.54,55 In the 1980s, Smith et al.56 showed that E. colidiarrhea in calves could be treated with phage, and this successful result then prompted the West to explore the possibility that phages could be used in human infections as well.
Because of the occurrence of multi-antibiotic-resistant bacteria, phages have been reappraised in the last two decades and are at the forefront again as therapeutic/prophylactic agents against human infectious diseases,18,42,57–60 aquaculture,15,61–63 agriculture, animal and plant pathogens,40,64,65 food23,36,66; wastewater31,67,68 and other subjects like biofilm removers69,70 and biosensor.71 Multiple studies on using phages in animal agriculture have explored Salmonella, E. coli, Clostridium, and Campylobacter for the pig, chicken, cattle, and sheep industries.22,72,73 Figure 1 summarizes phage applications in different areas.
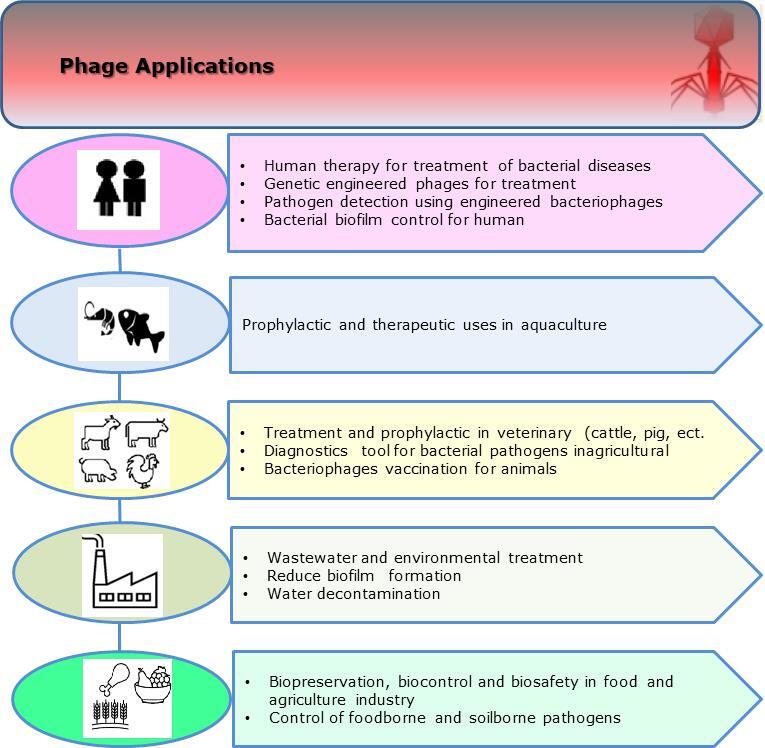
Figure 1.Illustration of phage applications in human, aquaculture, food, agriculture, and environment
Phage applications in fish diseases and Aquaculture
The aquaculture and farmed fish industry have high mortality rates and considerable economic losses because of certain microbial infectious diseases. Widespread bacterial fish diseases are aeromonasis, hemorrhagic septicemia, furunculosis, vibriosis, edwardsiellosis, mycobacteriosis, ulcer disease, columnaris, lactococcosis, enteric red mouth disease, fry syndrome, and cold-water disease, respectively.8 Phages were used to control and prevent bacterial infections caused by antibiotic-resistant bacteria at the laboratory level or in small-scale trials for field applications in aquaculture.
The essential step in aquatic phage therapy is identifying the fish disease agent and then detecting and isolating the phage that can effectively infect the host bacteria. The phage and bacteria interactions are essential to inactivate possible aquatic pathogens. Phages can interact with hosts bacteria to the lytic cycles, replicate their genome, and produce new phages that let out bacterial cell lysis into ponds, lakes, seas, rivers, and sewage, infecting new bacterial cells. New phages are exponentially replicated, and the number of bacteria decreases and disappears. Figure 2 is an overview of the steps of phage application in aquaculture.

Figure 2.Schematic steps on phage application in aquaculture.
1. Phages are isolated from ponds or aquatic animals 2. Phages are isolated, purified, and identified 3. Phage is added to the farm water/fish/hatchery 4. After adding phages to water, they attach and contact the infected bacterial pathogen and then undergo a lytic cycle. Phage DNA would penetrate the host bacteria and replicate, transcription, and translation. Then the phage would assemble, the fish pathogen would be lysed, and phages would be released from the pathogen bacteria 5. Lysis of fish pathogen bacteria 6. Results of phage therapy in fish diseases.
Our literature review screened numerous google scholar publications from 1997 to 2022 when typed in “Bacteriophages, aquaculture, and twelve fish pathogens” as the keywords (Figure 3). And as shown in the bar graph, the number of research studies on phage therapy applications in aquaculture has gradually increased worldwide for twelve bacterial agents.

Figure 3.Publication related to phage therapy in aquaculture over 25 years.
The bar indicates the number of the Web of Science search for publications related to phage therapy and genomes associated with the most important fish pathogens A. hydrophila, A. salmonicida, E. tarta, V. harveyi, V. parahaemolyticus, V. anguillarum, V. alginolyticus, F. columnare, F. psychrophilum, Y. ruckeri, L. Garviae, and S. iniae in the last 25 years.
There is a selection of studies on the genetic/morphologic identification, characterization of phages, and the effectiveness of different phages for biocontrol and treatment in eggs, larvae, juveniles and adult fish and shrimp, other aquatic organisms, and aquaculture food products.
In addition, numerous reviews have reported that the most studied phage families are Myoviridae, Podoviridae, and Siphoviridae as virulence and control tool against a wide variety of pathogens, A. salmonicida, A. hydrophila, E. tarda, Y. ruckeri, V. harveyi, V. parahaemolyticus, V. anguillarum, V. alginolyticus, F. columnare, F. psychrophilum, L. garviae and S. iniae, in vitro or in vivo.9,11,15,21,30,41,74–79
A list of reported phage applications and outcomes against the most significant bacterial pathogens in aquaculture from 1981 to 2022 is shown in Table 2.
Table 2.Morphology and genome characteristics of the fourteen phage families
Etiologic agent |
Disease |
Phage/Phages Cocktails |
Fish/shellfish/shrimp species |
Outcomes |
References |
Aeromonas salmonicida |
Furunculosis |
HER 110 |
Oncorhynchus fontinalis |
Use of bacteriophages has the potential to prevent of furunculosis in 3 days and to minimize the development of phage-resistant strains of A.salmonicida. |
Imbeault et al.26 |
|
Furunculosis |
O, R, B |
Salmo salar |
Phages were used orally, bath treatment and injection for therapy to A.salmonicida challenged fishes, but no protection was offered by any of the bacteriophage treatments. |
Verner-Jeffreys et al.80 |
|
Furunculosis |
PAS-1 |
O.mykiss |
Phage PAS-1 showed efficient bacteriolytic activity. In tank experiments, the administration of infected fish exhibited notable protective effects and increasing survival rates. |
Kim et al.81; 2015 |
|
Furunculosis |
AS-A |
Solea senegalensis |
Results showed that after 6 h of treatment the phage inhibited the growth of A.salmonicida both in batch cultures and seawater in the presence of fish juveniles. |
Silva et al.82 |
|
|
SW69-9, L9-6, Riv-10 |
Fish |
A new classifcation scheme for A.salmonicida phages. |
Vincent et al.83 |
|
Furunculosis |
AS-A, AS-D, AS-E |
- |
Phage cocktails developed phage cocktails reduced the population of A.salmonicida faster than single suspensions. |
Duarte et al.84 |
|
Furunculosis |
AS-szw, AS-yj, AS-zj, AS-sw, AS-gz |
- |
In vitro investigations into phages are prerequisite to obtain satisfying phage cocktails prior to application in practice. |
Chen et al.85 |
|
Furunculosis |
ASP-1 |
Carassius auratus |
ASP-1 phage was isolated and
characterized. Phage was stable over wide-range of temperatures, pH and salinity. ASP-1 showed 30 min of latent period, 16 PFU/infected cells of burst size and 40 min of rise period. |
Nikapitiya et al.86 |
|
Furunculosis |
vB_AsM_ZHF, ZHA, ZHD |
Scophthalmus maximus |
3 A.salmonicida subsp. masoucida phage isolates from sewage, and vB_AsM_ZHF exhibited the best antibacterial effect, based on in vitro sexperiment. |
Xu et al.87 |
A.hydrophila |
Motile Aeromonas, Hemorrhagic septicemia |
AH1 |
pond water |
First isolation of AH1 phage. |
Wu et al.88 |
|
Motile Aeromonas |
pAh1-C, pAh6-C |
Misgurnus anguillicaudatus |
Phages showed efficient bacteriolytic activity against fish-pathogenic A.hydrophila from loaches. The latent periods of the phages were estimated to be approximately 30 min (pAh1-C) and 20 min (pAh6-C). |
Jun et al.89 |
|
Motile Aeromonas |
φZH1 and φZH2 |
Oreochromis niloticus |
ΦZH1 and ΦZH2 administered via injection was found to be effective in treating fish infected with A.hydrophila shown through the significant decrease in number of A.hydrophila found in the water of treated fish. |
El-Araby et al. 2016 |
|
Motile Aeromonas |
pAh-1 |
Danio rerio |
pAh-1 as a lytic phage that strongly attacks the pathogenic A.hydrophila and higher survival rate of zebrafish. |
Easwaran et al.90,91 |
|
Motile Aeromonas |
AP1, AP2, AP3,AP4 |
O.niloticus |
Results achieved 94% elimination of A.hydrophila comparing to phage infectivity under basal conditions. In vivo efficiency of AP2 against A.hydrophila invading the aquaria of Nile tilapia was investigated. Elimination of A.hydrophila in the rearing water was detected after 24h. |
Hassan et al.92 |
|
Motile Aeromonas |
φF2, φF5 |
Pangasianodon hypophthalmus |
Phage treatments applied to bacterial strains during infestation of catfish resulted in the survival rates of the tested fishes, with up to 100% compared to 18.3% survival observed in control experiments. |
Le et al.93 |
|
Motile Aeromonas |
TG25P, CT45P |
P.hypophthalmus |
TG25P and CT45P were subjected to the phage cocktail to inactivate A.hydrophila. |
Hoang et al.94 |
|
Motile Aeromonas |
PVN02 |
P.hypophthalmus |
Confirmed that PVN02 is a novel lytic phage that could potentially be used as an agent to control A.hydrophila in striped catfish. |
Tu et al.95,96 |
|
Motile Aeromonas |
Akh-2 |
Misgurnus anguillicaudatus |
Isolated two phages that can infect A.hydrophila from seawater, isolation of more phages is promising, further isolation, characterization and application of A.hydrophila. |
Akmal et al.97 |
|
Motile Aeromonas |
MJG |
O.mykiss |
MJG had activity at temperature 10 °C between 60 °C and pH 2 to10, and its latent and rise periods were 30 and 40 min. MJG treatment would restore liver tissue damages and abolish the clinical signs of infection. |
Cao et al.98 |
|
Motile Aeromonas |
pAh6.2TG |
O.niloticus |
The pAh6.2TG was highly specific to A.hydrophila and infected 83.3% tested strains of MDR A. hydrophila (10 out of 12) with relative stability at pH 7-9, temperature 0-40◦C and salinity 0-40 ppt. |
Dien et al.99 |
|
Motile Aeromonas |
PVN02 |
P.hypophthalmus |
Without the existence of the phage, the highest mortality rate was 68.3 at the highest density of bacterial suspension and mortality rate at the highest density of bacterial suspension was significantly reduced to 8.33 ± 2.9% or 16.67 ± 2.9% at the phage dose of log 6.2 ± 0.09 or log 4.2 ± 0.09PFU/g. |
Dang et al.100 |
|
Motile Aeromonas |
AH-1, AH-4, and AH-5 |
Cerastoderma edule |
All phages were effective against A.hydrophila, but phage AH-1 (with a maximum reduction of 7.7 log colonies forming units CFU/mL. |
Duarte et al.101 |
|
Motile Aeromonas |
PZL-Ah1and PZL-Ah8 |
Aquatic amimal |
PZL-Ah1 and PZL-Ah8 were isolated and used to decrease infection. |
Yu et al.102 |
|
Vibriosis |
Ahy-Yong1 |
Cyprinus aka Koi |
It is stable at 30–40 ◦C and at pH 2–12. Ahy-yong1 revealed an effective biofilm removal capacity and an obvious protective effect in brocade carp. In vitro and in vivo experiments demonstrated ahigh antibacterial rate of Ahy-yong1 against A.hydrophila. |
Pan et al.103 |
A.hydrophila and P.fluorescens |
Motile Aeromonas |
50AhydR13PP, 60AhydR15PP, 25AhydR2PP, 22PfluR64PP, 67PfluR64PP, 71PfluR64PP, 98PfluR60PP |
O.mykiss |
The use of mixed phages increased the activity of lysozyme, total protein and immunoglobulin level. Ceruloplasmin level in the fish serum remained unchanged. Killing and metabolic activity of spleen phagocytes and proliferation of pronephros lymphocytes were higher compared to the control group. |
Schulz et al.104 |
A.punctata |
Septicemia, diarrhea, wound infections |
IHQ1 |
Stream water |
Characterization of phage IHQ1 showed that it was very efficient in lysing A.punctata, combined with its outstanding thermal and pH stability; |
Haq et al.40 |
A.hydrophila and
Edwardsiella tarda |
Hemorrhagic septicaemia, Edwardsiellosis |
A1,A2,E1,E2,T1,T2 |
Anguilla japonica |
Phages decreased the bacterial host after 2 hours. In pond water, phage treatment reduced 250-fold the A.hydrophila population in 8 h, while phage population increased |
Hsu et al.105 |
E.tarda |
Edwardsiellosis |
G1, G7, G8, G9.2 |
P.hypophthalmus |
Phages latent period were 55-70 min and 28-160 PFU/cell. E.ictaluri was challenged in vitro in broth and was inactivated by single phage for 18-20 h. |
Hoang and Pham94 |
|
Edwardsiellosis |
ET-1 |
Anguilla japonica |
Phages for phage typing of E.tarda could not be found because175 strains of E. tarda used in this study were divided into 15 phage types by 8 strains of phages and 87 strains of E.tarda were not sensitive to the phages. |
Wu and Chao106; Yamamoto and Maegawa107 |
|
Edwardsiellosis |
ETP-1 |
D.rerio |
Zebrafish was bath exposed for 12 days to phage and challenged with E.tarda, the survival rate in 4 days. |
Nikapitiya et al.108 |
|
Ascites |
PETp9, PVHp5 |
Scophthalmus maximus |
The results showed that the abundance of Vibrio species and Edwardsiella species in turbot's intestine was significantly reduced by feeding with phage cocktails of E.tarda phage PETp9 and V.harveyi phage PVHp5. |
Cui et al109 |
|
Edwardsiellosis |
phage |
P.olivaceus |
E.tarda phages were identified in the seawater before the disease outbreak and during the disease prevalence, but not detected after the outbreak terminated. |
Matsuoka and Nakai110 |
E.ictaluri |
Septisemia |
φFeiDWF,φ FeiAU, φFeiMSLS |
Channel catfish |
Three E.ictalurispecific bacteriophages isolated from geographically distant aquaculture ponds, at different times, were sequenced and analyzed and these bacteriophages are lytic and can be used in infection diseases. |
Walakira et al.111; Carrias et al.112 |
E.piscicida |
Edwardsiellosis |
vB_EpM_ZHS, vB_EpP_ZHX |
S.maximus |
Cocktail phage significantly inhibited bacterial growth in vitro and decreased approximately 40% of mortality rate and an order of magnitude of bacterial burden in zebrafish and turbot infected by E.piscicida. |
Xu et al.113 |
Vibrio sp. |
Vibriosis |
ValLY-3, VspDsh-1, VspSw-1,
ValSw4-1,VpaJT-1, |
Litopenaeus vannamei |
Phage cocktail preparation showed in vitro higher e ciency in inhibiting the growth of Vibrio sp. Va-F3 than any single phage. |
Chen et al.114 |
Vibrio harveyi |
Luminescent vibriosis |
Viha8, Viha10, Viha9, Viha11 |
Penaeus monodon |
Phage Viha10 was effective in reducing the population of V.harveyi in the biofilm and application of phages Viha8 and Viha10 resulted in 85% survival of larvae |
Karunasagar et al.115 |
|
Vibriosis |
Viha 1, Viha 2, Viha 3, Viha4, Viha 5, Viha 6, Viha 7, |
Penaeid shrimp |
Six phages were highly lytic for V.harveyi and they were potential candidates for biocontrol of this bacterium. |
Shivu et al.116 |
|
Vibriosis |
Siphoviridae |
P.monodon |
The study concluded tha tbacteriophage has the potential in management of luminous vibriosis in aquaculture. |
Vinod et al.117 |
|
Vibriosis |
VH1, VH8 |
Shrimp |
All the isolates of phage caused lysis of the host bacteria within 2 hours. |
Srinivasan et al.118 |
|
Vibriosis |
VHM1, VHM2, VHS1 |
P.monodon |
Post larval stages of shrimp were treated with bacterium (105 cells/mL) first in laboratory trials followed by single phage treatment about 109 PFU/mL) and phage cocktail treatment about 109 PFU/mL. It can be used as a potential alternative treatment for the control V.harveyi in shrimp |
Stalina and Srinivasan119 |
|
Vibriosis |
VhCCS-01, -02, -04, -06, -17, -20, -19, -21 |
Panulirus ornatus |
The lytic ability of 6 phages suggested that they are appropriate for phage therapy. |
Crothers-Stomps et al.120 |
|
Vibriosis |
PVHp5, PVHp8 |
S.maximus L. |
Two phages are isolated and feeding phage cocktails may be another optimal therapeutic agent against V.harveyi infection in turbot |
Cui et al.121 |
|
Vibriosis |
PW2 |
Shrimp |
Phage performanse depends on temperature and pH. Phage adsorption rate increased rapidly in15 min of infection to 80% and continued to increase to 90% within 30 min of infection. |
Phumkhachorn and Rattanachaikunsopon122 |
|
Vibriosis |
VHLM |
P.monodon |
Phage showed a narrow host range and an apparent preference for V.harveyi rather than other 63 isolates and 10 other. |
Oakey and Owens123 |
|
Vibriosis |
vB_VhaS-a, vB_VhaS |
Haliotis laevigata |
Threatment with phages resulted in 70% of survival. |
Wang et al.124 |
V.parahaemolyticus |
Luminescent vibriosis |
pVp-1 |
Oysters |
Bath immersion and surface-application of the lytic phage effectively reduced the bacterial growth of V.parahaemolyticus. |
Jun et al.125,126 |
|
- |
vB_VpS_BA3, vB-VpS_ CA8 |
Sewage |
In the in vitro phage trial CA8 had the potential for phage therapy. |
Yang et al.127 |
|
Vibriosis |
A3S and Vpms1 |
Litopenaeus vannamei |
Phages were reduce the mortality rates of larvae caused by V.parahaemolyticus, especially when applied at the early stage (6 h post-infection). |
Lomelí-Ortega and Martínez-Díaz128 |
|
Vibriosis |
ФVP-1 |
Penaeid shrimp |
Ability to infect V.parahaemolyticus and V.alginolyticus and showing also biofilm reducing capacity. |
Matamp and Bhat129 |
|
Vibriosis |
VPp1, VP-1, VP-2 and VP-3 |
Oysters |
V. parahaemolyticus in oysters, which decreased by 2.35–2.76 logCFU/g within 36 h. |
Rong et al.130; Mateus et al.131 |
|
Vibriosis |
VP93, VpV262 |
- |
Phage growth can be modelled if phage-sensitive and resistant cells that convert to each other with a high frequency are present in clonal cultures of pandemic V.parahaemolyticus. |
Bastías et al.132 |
|
Vibriosis |
AMN2, FT2, FT3, KD1,V1, AMN1, AMN3, PL1, V2, V4,
V5 and V6 |
Litopenaeus vannamei |
Phage application against V.parahaemolyticus in shrimp showed 78.1% reduction in bacterial counts within 1 h. |
Dubey et al.133 |
|
Vibriosis |
VP1, VP7 and VP |
P.monodon |
The cumulative survival rate were 70% after 144 h and others 60–65% . |
Alagappan et al.134 |
|
Vibriosis |
PVP1, PVP2 |
Apostichopus japonicus |
Feeding phage cocktails might be another optimal therapeutic agents to treat V. parahaemolyticus infections in sea cucumber aquaculture. |
Ren et al.135 |
V.anguillarum |
Vibriosis |
AS-1 |
Fish |
Diseases controlled and efficacy of plating |
Pereira et al.10 |
|
Hemorrhagic
septicemia |
ALMED, CHOED, ALME, CHOD, CHOB |
Salmo salar |
Phages infect both V.anguillarum, V.ordalii but not V.parahaemolyticus, CHOED phage protect fish against experimentally induced vibriosis |
Higuera et al.136 |
|
Vibriosis |
PVc-1, PVc-2 |
Dicentrarchus labrax |
Genomic characterization were made by looking at genome size |
Cagatay137 |
|
Vibriosis |
KVP40 |
Gadus morhua L. and S.maximus L. |
Phage decreased mortality of cod and turbot larvae in experimental challenge assays with V.anguillarum pathogens suggested that phages can reduce Vibrio mortality in turbot and cod larvae. |
Rørbo et al.138 |
|
Vibriosis |
VP-2, VA-1 |
D.rerio |
Phage therapy is a suitable alternative approach against vibriosis in Zebra fish larvae. |
Silva et al.139 |
V.alginolyticus |
Vibriosis |
φSt2 and φGrn1 |
Marine fish, live feeds (Artemia) |
Phage cocktail live prey A.salina, led to93% decrease of Vibrio population after 4 h of treatment in fish hatcheries. |
Kalatzis et al.140 |
|
Vibriosis |
VEN |
- |
These results suggest that VEN may provide a good candidate to control recurrent diseases caused by V.alginolyticus. |
Kokkari et al.141 |
V.coralliilyticus |
Coral diseases |
YC |
Acropora millepora |
Phage has isolated and identified a effective against the coral pathogen V.coralliilyticus |
Cohen et al.142 |
|
Mortality of larvae |
pVco-14, pVco-5, pVco-7. |
Crassostrea gigas |
Higher survival rate in phage-treated oyster larvae |
Kim et al.143,144 |
V.splendidus |
Skin ulcer |
vB_VspP_pVa5 |
Fish |
The phage showed a huge bactericidal activity and proposed as potential phage cocktails and suitable for the biological control of V.splendidus. |
Katharios et al.145 |
Photobacterium damselae formerly Vibrio damselae |
Opportunistic pathogens |
vB_Pd_PDCC-1 |
Seriola rivoliana |
vB_Pd_PDCC-1 against P. damselae subsp. damselae was isolated and characterized. vB_Pd_PDCC-1 increased the hatching rate of eggs, and reduced presumptive bacterial species |
Veyrand-Quir et al.146 |
Pseudomonas plecoglossicida |
Hemorrhagic ascites |
PPpW-3, PPpW-4 |
Plecoglossus altivelis |
Mortalities of fish receiving PPpW-3, PPpW-4, PPpW-3/W were 53.3, 40.0, 20.0 and 93.3%, respectively when phage impregnated feed was used to ayu with disease decreased after a 2 wk period. |
Park and Nakai61; Kawato et al.147 |
Pseudomonas aeruginosa |
Aeromonas infection |
Pseudomonas phage |
Clarias gariepinus |
First report of application of phage therapy against MBL producing P.aeruginosa isolated from aquatic ecosystem |
Khairmar et al.148 |
Lactococcus garvieae |
Lactococcosis |
PLgT-1 |
Marine fish |
The lysogenic phage PLgT-1 may be involved in the transfer of a virulence factor into L.garvieae strains colonizing marine fish in Japan |
Hoai and Yoshida149 |
|
Lactococcosis |
PLgY-16, PLgY-30, PLgW-1 |
Seriola quinqueradiata |
Phage administered either intraperitoneally or orally protected fish from L.garvieae infection. |
Nakai et al.150; Park et al.151,152 |
|
Lactococcosis |
PLgY-30 |
S.quinqueradiata |
The complete sequence of L.garvieae phage PLgY-30 was obtained |
Hoai et al.153 |
|
Lactococcosis |
PLG-II |
S.quinqueradiata, S. dumerili, S. lalandi |
Genomics analysis suggests that phage PLG-II might represent a novel species in the genus Uwajimavirus. phage PLG-II a suitable candidate for control of L.garvieae serotype II fish infections. |
Akmal et al.154 |
|
Lactococcosis |
WWP-1 |
O.mykiss |
Phage WWP-1 represented optimal antibacterial activity at temperatures ranging from 15 to 30 C, suggesting that it could be very effective at rainbow trout rearing temperature. In vivo experiment result, WWP1 could decrease mortality rate of infected rainbow trout in aquaculture. |
Ghasemi et al.155 |
Streptococcus iniae |
Streptococcosis |
PSiJ-31, PSiJ-32, PSiJ-41, PSiJ-42, PSiJ-51, PSiJ-52 |
Paralichthys olivaceus |
Fish were injected intraperitoneally with bacteria and 1 h later IP-injected with a mixture of two or four phage isolates and observed at 25°C for 2 wk. Mortalities of fish receiving phages were significantly lower than those of control fish without phage-treatment. |
Matsuoka et al.156 |
Yersinia ruckeri |
Enteric redmouth disease, yersiniosis |
ɸ 2, ɸ 3, ɸ 3, ɸ 9, |
S.salar |
4 different phages and a cocktail with a combination of the four was tested. Non-vaccinated fish had no phage reactive
antibodies but inactivated phages were highly immunogenic for salmon and a good specific anti-phage antibody response was obtained in immunized salmon. |
Strand157 |
|
Enteric redmouth disease, yersiniosis |
YerA41 |
Salmonid fish |
YerA41 genome sequence were determined, we performed RNA sequencing from phage cells at different time infection. |
Leskinen et al.158 |
|
Enteric redmouth disease, yersiniosis |
φNC10 |
O.mykiss |
The φNC10 associated polysaccharide depolymerase activity reduced the ability of Y.ruckeri cells to cause mortality following intraperitoneal injection into fish. Potential usage of φNC10 for Y.ruckeri infection. |
Welch159 |
Flavobacterium columnare |
Columnaris disease |
FCP1, FCP9, FCP1 |
Clarias batrachus |
Phage treatment led to disappearance of gross symptoms, negative bacteriological test, detectable phage and 100% survival in experimentally infected C.batrachus that was treated with a virulent bacteria and FCP1 a significant decrease in fishes. |
Prasad and Kumar160; Prasad et al.161 |
|
Columnaris disease |
FKj-2, FL-1, FCL-2, FCV-1 |
O.mykiss, D.rerio |
Phages infecting F.columnare were isolated only from fish farms during disease outbreaks. 100% of the zebrafish and 50% of the rainbow trout survived in the phage treatment. |
Laanto et al.162,163 |
|
Columnaris disease |
FCO-F2 to FCOV-F2, FCOV-F5, COV-F25, FCO-F9 to FCL-2, FCOV-F13, FCOV-F45 |
Salmonid |
Bacterial infection decreased in the exposure cultures but started to increase after 1 to 2 days, along with a change in colony morphology from original rhizoid to rough. |
Kunttu et al.164 |
|
Columnaris disease |
PFlc-1 ve PFlc-2 |
Carassius auratus |
Genomic characterization were made by looking at genome size |
Cagatay165 |
|
Columnaris disease |
FCOV-S1 to 62 |
aquaculture environments |
Examined phenotypic and genetic characteristics 63 phages from fish farms in Finland and Sweden. |
Runtuvuori-Salmela et al.166 |
F.psychrophilum |
Rainbow trout fry
syndrome, bacterial
coldwater disease |
FpV-1 to FpV-22, FpV2, FpV4,
FpV7, FpV9, FpV10,FpV14, FpV19 |
O.mykiss |
Phages with strong lytic potential against F.psychrophilum host strains thus provided the foundation for future exploration of the potential of phages in the treatment of both diseases. |
Stenholm et al.167 |
|
Rainbow trout fry
syndrome |
PFpW-3, PFpC-Y, PFpW-6, PFpW-7,PFpW-8 |
Plecoglossus altivelis
altivelis |
Among the phages, in in vitro assays, PFpW-3 displayed high infectivity for F. psychrophilum isolated from ayu fish, indicating that it could have treatmentment of diseases |
Kim et al.168 |
|
bacterial
coldwater disease |
1H, 6H, 9H, 2P, 23T, 2A,FpV4, FpV9 |
S.salar, O.mykiss |
15 bacteriophages able to infect some of the F.psychrophilum isolates and characterized six of them in detail. Phages were injected i.p. in a ratio of 10:1 (PFU: CFU) and significantly decrease fish mortality. |
Castillo et al.169 |
|
Rainbow trout fry
syndrome |
FpV-4 and FpV-9 |
O.mykiss |
Diet with phage additives might be a method for delivery of phages to F.psychrophilum-infected fish. the potential of phages to spread to inner organs of rainbow trout after i.p. injection and toproliferate and maintain infectivity for up to 10 days. |
Madsen et al.170 |
|
Rainbow trout fry
syndrome, bacterial
coldwater disease |
FpV-9 |
O.mykiss |
Survival of FpV-9 in vivo in juvenile fish after by bath, oral intubation into the stomach and phage-coated feed. Phages via coated feed pellets constitutes a promising method of treatment and prevention of diseases. |
Christiansen et al.171 |
|
Rainbow trout fry
syndrome |
FpV4 and FPSV-D22 |
O.mykiss |
The delivery of phages to fish organs by oral, suggests that higher phage dosages on feed pellets to offer fish an adequate protection against F.psychrophilum infections. |
Donati et al.172,173 |
The result of summarized studies in Table 2 using phages specific to 12 fish diseases agents as direct or suspensions of single or cocktail, oral administration, injections, or as aquafeed174–176 recommend that phages could be beneficial to prevent and treat bacterial infections of aquatic animals. Even though different methods are used, the literature indicates that the most prophylactic impact appears when administered intraperitoneally (Americo et al., 2020). Additionally, in recent years, the other practical way was using commercial phages developed and used against some pathogens of aquaculture.41,177 For example, Intralytix and Phage Biotech Ltd have developed phages to destroy Vibrio spp. in oyster and shrimp aquaculture,178,179 Phage named BAFADOR® registered by Proteon pharmaceutical against Aeromonas spp. and Pseudomonas spp.180,181 ACD Pharma has developed phage solutions against Y. ruckeri.182 Fixed Phage Ltd. has developed aquafeed-phage pellets.183 Mangalore Biotech Laboratory has also developed LUMI-NIL MBL to control pathogens in shrimp.184
The first research on phage therapy used in aquaculture was notified by Wu and co-workers in 1981 as the pathogenicity loss of A. hydrophila to loach (M. anguillicaudatus), in which Ah1 phage infected the pathogen.88 Numerous studies have declared the accomplished use of more than 35 A. hydrophila phages aiming to control motile Aeromonas and septicemia from 1981 to 2022 (Table 2).40,90,92,93,96–105,175,185,186
Around 22 phages with treatment activity against A. salmonicida (caused furunculosis) have been identified and characterized from farm fish (Table 2).26,80–87
Four phages named PLgY-16, PLgY-30, and PLgW-1 were used to treat L. garvieae infection in yellowtail (S. quinqueradiata)150,151(p1998) and P. plecoglossicida infection in ayu (P. altivelis)61,152 in the 1990s.
From 2000 to 2022, more lysogenic phages named PLgT-1, PLgY-30, PLG-II, and WWP-1 were involved in L. garvieae infection colonizing marine fish, S. quinqueradiata, S. dumerili, S. lalandi, O. mykiss (Table 2).149–155
Several phages for controlling F. columnare, which causes Columnaris disease in fish like Clarias batrachus and O. Mykiss, were isolated genetically and characterized.166
Application of columnar phages (FCP1, FCP9, and FCP1) to infected fish resulted in the resolution of disease symptoms and stopped infection.160,161 Four phages infecting F. columnare were isolated from fish farms during columnaris outbreaks. The zebrafish (100%) and the rainbow trout (50%) survived after the phage treatment.162,163 Furthermore, several studies reported isolation and application for F. psychrophilum phages that could be used for biocontrol of the fry syndrome and cold water disease in S. salar, O. Mykiss, and P. altivelis. Lytic phages against F. psychrophilum strains provided the future potential in the treatment of this disease (Table 2).167–173
The therapeutic effects of six S. iniae lytic phages with dsDNA were studied against Streptococcosis infection in P. olivaceus at 25°C for 2 weeks (Table 2).156
Y. ruckeri is the causative bacterium of yersiniosis, known as enteric red mouth disease in freshwater salmonid fish. Yer A41, ɸ 2, ɸ 3, ɸ 3, ɸ 9 and φNC10 phages were tested as a combined or single intraperitoneal injection to treat Y. ruckeri and antibody production was reported in phage-treated fish (Table 2).157–159
Two phages, named PT2 and phiKMV, were obtained from sewage, identified, and treated for P. aeruginosa infection at the surface of C. gariepinus. It was observed that the number of infective lesions decreased after 8-10 days in phage-treated fish.148
Similarly, phages, namely PPpW-3 and PPpW-4, were used to treat bacterial hemorrhagic ascites disease caused by P. plecoglossicida in P. altivelis.61,147
There are a few studies reported that specific phages of E. tarda, E. İctaluri, and E. piscicida stop especially growth of bacteria and reduce edwardsiellosis in vitro in D. rerio, P. hypophthalmus, A. japonica and S. maximus.94,106–113
Various Vibrio species, such as V. harveyi, V. vulnificus, V. anguillarum, V. parahaemolyticus, V. alginolyticus, V. coralliilyticus, V. splendidus, and P. damselae (formerly V. damselae) are the cause of vibriosis have also been controlled by vibriophages which is biocontrol agents in fish (S. maximus L., S. salar, D. labrax, D. rerio, G. morhua L.) and P. monodon, L. vannamei, P. ornatus, H. laevigata, A. japonicus.187 Studies have reported that approximately 60 bacteriophages were morphologically identified and genome sequenced and applied against Vibrio strains with no side effects shown in Table 2.10,114–118,121,127–131,134,138,140,145,146,165
Conclusion
Despite good management practices, chemotherapeutic and prophylactic applications such as vaccines and various antibiotics for producing fish, crustaceans, and mollusks in many countries where aquaculture is a vital economic resource, bacterial diseases still stay a severe problem as they cause high mortality rates. An additional problem of bacterial infection is nowadays not only bacterial resistance to antibiotics but also the use of all known antibiotics in the treatment. Therefore, phage therapy has been shown as a perfect and valid option for antibiotic treatment. It is also an environmentally friendly, relatively rapid, simple administration, and inexpensive approach to disease prevention and control in aquaculture. Furthermore, therapeutic and prophylactic phage applications in aquaculture can effectively inactivate and eliminate pathogenic bacteria without harming useful microbiota and are easy to apply at various stages of vertebrates or invertebrates. Most of the aquaphage studies of the last 40 years, which we reviewed in this article, showed us that phage therapy has a general protective effect and can be substituted for possible antibiotics (Table 2). From the author’s perspective, although phage applications provide an optimistic view of future benefits for disease prevention and treatment in the world aquaculture sector, caution is required as the potential evolution of phage resistance against bacterial agents may also be present. In addition, more field applications should be made with large-scale cultivation and long-term preservation, and standardized methods and formulations should be developed. Furthermore, new commercially patented aquaphage products must be developed for future aquaculture practice. More research and improvement in phage therapy will play a significant role in sustainable aquaculture globally.
Author contributions
Conceptualization: Ifakat T. Çağatay (Lead). Investigation: Ifakat T. Çağatay (Lead). Writing – review & editing: Ifakat T. Çağatay (Lead).